More than 300 molecular species have been identified in space, primarily through radio astronomy (about 90%) using to their rotational spectra. However, radio astronomy has sensitivity limits, especially for large symmetric molecules such as C60. For these molecules, we use their vibrational (IR) and/or electronic (near-IR/visible) spectral signatures. Among the 600+ diffuse interstellar bands (DIBs), absorption bands ranging from the UV to the near-IR, only 4 have been unambiguously assigned to C60+, while the others remain unexplained.
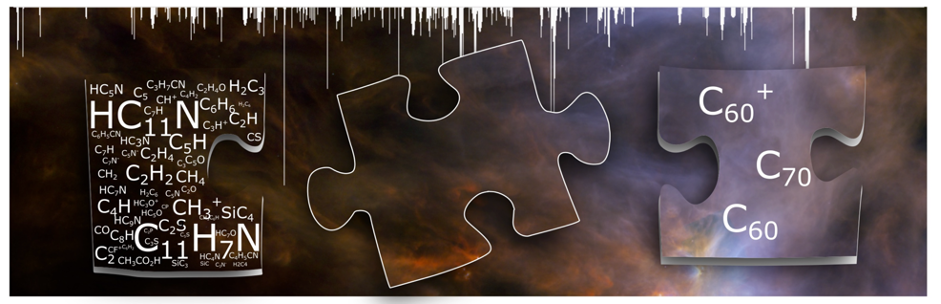
The mystery of the DIBs highlights the need for reliable experimental data on large charged molecules. Three large molecules (C60, C60+, and C70) have been detected via their IR/near-IR signatures, but the next largest identified molecules contain only 17 carbon atoms (cyanopyrene). Solving this mystery would enhance our understanding of the carbon life cycle and stellar chemistry, which is essential for modeling complex chemical processes and the condition necessary for the emergence of life.
We aim to push the boundaries of experimental methods for the spectroscopic study of molecular ions, which are essential for understanding phenomena such as diffuse interstellar bands and unidentified infrared bands. Studying ionic molecules has proven challenging due to their high reactivity and transient nature. We seek to play a key role in advancing this field by producing gas-phase vibrational and electronic spectra that can be directly compared to astronomical data. We have developed a unique experimental setup, enabling significant progress in the detection of large molecular ions in space, surpassing the capabilities of radio astronomy.
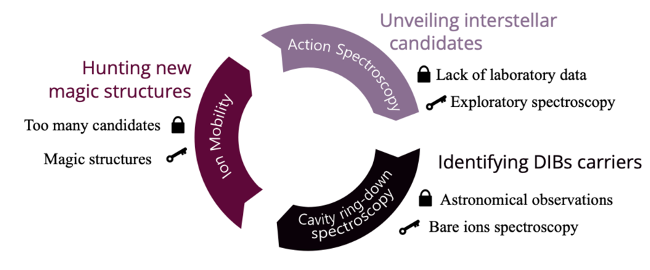
Our experimental approach is based on three steps, as schematically illustrated in the Figure above. The first step, which relies on mass spectrometry and ion mobility techniques, will identify promising candidates based on their thermodynamic properties and formation kinetics in a laser ablation source that mimics stellar condensation. The second step involves using action spectroscopy in an exploratory manner to further refine the selection of promising candidates. The final step consists of recording their spectra using cavity ring-down spectroscopy (CRDS) or two-color resonance-enhanced photodissociation (REPD) spectroscopy, so that the spectral data obtained in the laboratory can be directly compared to astronomical observations—something that remains a major challenge today. The experimental setup is schematically represented in the figure below.
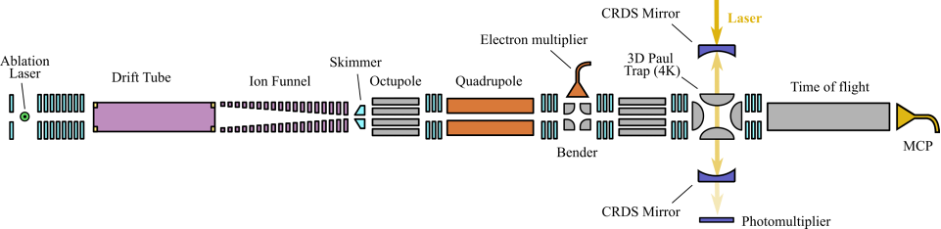
In addition, when we aim to record spectra using CRDS on small- or medium-sized ions, we use an experimental setup that couples a supersonic jet with an electrical discharge, where the CRDS is performed just downstream of the discharge. A time-of-flight system is also incorporated into this setup to optimize the formation conditions of the ions of interest, as shown in the figure below.
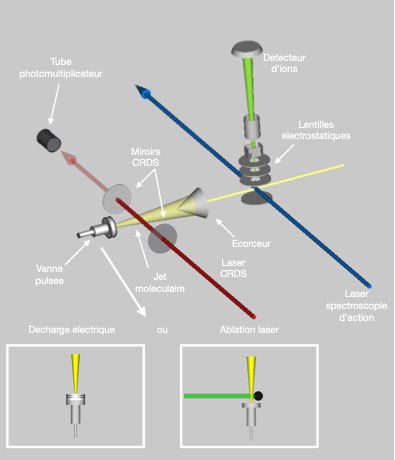
Contacts:
Stéphane Douin
Bérenger Gans
Ugo Jacovella